Total food grain production on global basis has shown a leap from 500 to 700 million tons since the end of 20th century till now (FAO, 2018). International food demand is estimated to rise from 70 to 100% in the coming decades to cope with the rising human population (Foong et al., 2020). India produces about 250 million tons of grain per annum, out of which about 11-15% is lost annually due to pests and other causes (Walter et al., 2016). To avoid/minimize losses, pesticides are being used that deter, discourage, incapacitate or kill certain pest populations viz., insects, fungi, rodents, weeds etc., and are indispensable to modern agricultural production and important for ensuring national food security. Pesticide production worldwide has increased from 0.2 million tons in 1950s to more than 5 million tons by 2000 (11% increment per year). According to an estimate, 5.2 billion pounds of pesticides are used worldwide every year. In India, total pesticide consumption has shown a variable trend from 1980s till 2017, as presented in Fig. 1 (Indiastat, 2019). Under field conditions, only 0.1% of pesticide sprayed reaches the target pests, and the rest 99.9% goes into the environment, thereby adversely affecting and contaminating the ecosystem and its components like air, water and soil in major (Pimentel, 1995). However, according to Parte et al. (2017b), only 10% of pesticide sprayed reaches the target organism. The rest of it is deposited on/in non-target areas, resulting in the pollution of soil, water, air etc. Furthermore, the metabolites formed out of pesticide degradation persist in the environment for a longer period e.g. organochlorines (OC). Szeto and Price (1991) recovered the residues of OC from aquatic bodies at the detection limit even after 20 years of ban. Some metabolites are even more toxic than the parent compounds (Rasool et al., 2021, 2022). Among the OC’s, dichlorodiphenyltrichloroethane (DDT) being most persistent has entered into the various food chains operating in an ecosystem and underwent biomagnification due to its indiscriminate use. The use of pesticides above the recommended dose is perilous to the environment, deteriorates the fertility of the soil and also has adverse effects on all living organisms (Parte et al., 2017b). Also, there have been concerns about the pesticide in context to the occupational hazards, residues in food and water above the permissible limit even though these are manufactured under strict regulation to function with certainty and minimal effect on non-target organisms (Damalas and Eleftherohorinos, 2011). There are reports of exposure of non-targent organisms like pollinators to pesticides resulting in economic and ecological losses (Brar et al., 2022). So, it becomes important to have vast knowledge about the nature of pesticide to be applied, their movement in soil and various tactics to prevent their risk to non- target organisms. In this review, information on pesticide interactions, their mobility, degradation and risk of contamination are discussed. |
Pesticide residues The pesticides may enter into the given environment through several ways namely; soil, water and air (Fig. 2). Once the soil application of pesticides is done, it enters the various soil medium components after dissolving rapidly (Usman, 2018). When applied to crops, the foliar pesticides also enter the soil through various routes, mainly the drift losses and wash off due to rains, thereby polluting the water bodies. The active ingredient of soil-applied pesticide granules reaches directly into the soil environment through leaching. The movement of the pesticide in soil is further affected by soil structure, irrigation, pesticide formulation, soil moisture/water content, pesticide application time and rainfall (Flury, 1995). The pesticides contaminate the soil and accumulate in the crops sown. Various forms of pesticide residues i.e. free and bound, are formed as a result of pesticide degradation. The free residues are readily extractable from the soil without change in chemical structures. However, bound residues are resistant ones for extraction. The bound residues are defined as “chemical species originating from pesticides, using good agricultural practices and are non-extractable by methods which do not significantly change the chemical nature of these residues” (Roberts, 1984). Because of their non-extractability, these have environmental significance (Khan, 1982). The bound residues are further of two types: available and non-available. The distinction has been made by Khan (1982) as: the available fraction of bound residue is easily available to plants and soil microfauna, while the non-available fraction cannot be tapped by these organisms. Thus, the study of pesticide dynamics i.e. whether the pesticide has degraded, absorbed, adsorbed or transformed, has become important (Lopez-Blanco et al., 2005). According to U.S. Environmental Protection Agency (USEPA), water has more contaminants from organochlorines and organophosphate groups, and their concentration is higher at surface level of water than in groundwater, and humans are at maximum risk of getting exposed to pesticides by means of water contaminated with these toxic compounds (Younes and Galal-Gorchev, 2000). Soil-pesticide interactions Adsorption is the important phenomenon with which the pesticide interacts with the soil particles. The extent of adsorption is controlled by various factors involving the properties of soil namely; texture, structure and pesticide too i.e. its chemical functions, molecular structure, acid-basic nature, solubility, polarity (Pignatello and Xing, 1996). Adsorption may be physical or may be due to weak interactions like electrostatic or van der Waals forces. The pesticide persistence in soil depends on the stable linkages between unaltered pesticides or their metabolites (Dec and Bollag, 1997). The compounds and their metabolites which are adsorbed onto the soil (through cation exchange or ionic bonding) exist as cations or get protonated to become cationic. The ionic bonding is due to easily ionisable or ionized humic substance containing carboxylic and phenolic hydroxyl groups e.g., the paraquat and diquat (bipyridilium pesticides) form stable bonds with the carboxyl groups through ion exchange. On the other hand, binding of amitrole and triazine herbicide which are less basic in nature are affected pH (Weber et al., 1969). From toxicological point of view, the xenobiotics binded to stable soil compound i.e. humus leads to: (a) decrease of availability of material to the biota (b) reduced compound toxicity (c) immobilisation of the compound thus reducing transport and leaching properties (Dec and Bollag, 1997). Factors affecting fate/ movement Adsorption-desorption phenomena strongly affect pesticide behaviour in soil. For accurate prediction of mobility and bioavailability of the chemicals, it is important to know the processes influencing the soil solution composition, so as to reduce or limit their impact on the ecosystem and non-target organisms. Pesticide leaching phenomenon and hence the risk to groundwater contamination depends on factors which are broadly classified into three main categories as pesticide properties, properties of soils and miscellaneous factors (Fig. 3). Pesticide properties Water solubility: It pertains to the pesticide amount that will dissolve in a known amount of water, and its units of measurement are milligrams per liter of water (mg L-1) or parts per million (ppm). The water solubility of pesticides affects its potential to leach down the soil as the seeping water carries down the water-soluble chemicals. Therefore, the pesticides having high hydrophilicity are more soluble and likely to be carried away with runoff water and contaminate the groundwater. On the other hand, the fate of poorly soluble pesticides not incorporated into soil is decided by the runoff water or erosion (Geyikci, 2011). Volatility: The pesticides which remain exposed to atmosphere are likely to be lost in the form of gas and the process is known as volatilization. More the tendency of a pesticide to become a gas, more are the chances of escaping into the environment. Loss of pesticides through volatilization is insignificant for most pesticides compared to leaching or surface losses. Various environmental factors such as temperature, humidity and aerial drift affect the volatilization of pesticides. A highly volatile pesticide has the least water solubility, thus has more possibility for escaping into the atmosphere, and a very low amount is leached to groundwater. Volatility may account for a significant pesticide dissipation pathway depending upon the environmental conditions, leading to a loss of up to 90% of the applied pesticide. Such losses have been reported in the case of trifluralin, which dissipated to 90% within a week of application by means of volatilization. Further, the gaseous form of pesticides in the air is a potential water contaminant that may get drifted from air. To reduce the volatilization losses, surfactants or carriers can be put into use (Geyikci, 2011). Adsorption: Literally, adsorption is a phenomenon of binding of a substance onto a solid surface. Here, in the context of the review, it refers to the pesticide binding to soil particles. The amount of pesticide adsorbed depends upon the soil properties such as texture, structure, pH, and the type of pesticide applied. Soils with high clay content or high organic matter adsorb pesticides strongly. This strong bond formation further affects the availability of pesticides to be taken up by plants, to get leached and volatilized (Tiryaki and Aysal, 1999). But this strong bond cannot resist the runoff water, and the soil particles and pesticides adhered are easily eroded away. In contrast, loosely bound pesticides with high water solubility will get easily leached to varying degrees. A term called adsorption partition coefficient (Kd) has been proposed for a pesticide’s potential to get adsorbed onto soil particles. The partition coefficient and leaching potential share a reciprocal relationship as the lower the value of partition coefficient, greater is the pesticide leaching potential (Geyikci, 2011). Degradation: By the process of photodecomposition, microbial degradation and various other chemical and physical processes, the pesticide is broken down into different chemical forms. Pesticide degradation in soil, i.e. post-volatilization and photo-degradation, is considered an important process in determining the fate of pesticide (Hester and Harrison, 2017). The rate of pesticide degradation defines its persistence in the soil, which in turn affects its leaching potential. The advance prediction of both rates and routes of pesticide degradation is difficult. Degradation products are more polar than parent pesticide but less biologically active Hence, they are more prone to leaching. In contrast to this statement, the biological oxidation products of aldicarb retain their biological activity (Nicholls, 1987). Sharma and Singh (2013), while studying the metabolism of imidacloprid in soil, reported the formation of nitrosamine and 6-chloronicotinic acid. Other metabolites of imidacloprid in soil like imidacloprid-nitrogunidine, olefin, urea have also been reported, out of which olefin and nitrosimine are having more insecticidal activity than parent compound, and guanidine is having more mammalian toxicity (Sahoo and Singh, 2016). Some neonicotinoid pesticides along with their fate in soil are shown in Fig. 4. Properties of soils Organic matter: The soil organic matter is directly correlated to the chemical adsorption. With increase in soil organic matter, adsorption also increases, thus, very less amount is available for degradation in the soil, and it is usually interrupted by a continuously increasing microbial population that results in increased pesticide degradation (Nicholls, 1987). Clay content: Clayey soil has more than 40 per cent of clay particles and has large internal reactive surface area, thus facilitating more pesticide adsorption. Data generated from research has shown a direct correlation between the clay content in the soil and their binding ability to retain pesticides (Hermanson and Forbes, 1966). This correlation is a bit complex, because clay is in the form of colloids in the organic matter. Following are the factors influencing pesticide adsorption by soil colloids (Bailey and White, 1970): i) physicochemical configuration of the colloids ii) pesticide physicochemical configuration iii) pesticide dissociation constant iv) pesticide water-solubility v) size of the pesticide molecule vi) hydrogen ion concentration of soil vii) temperature conditions viii) the moisture content of the soil ix) electrical potential of clay surface and x) pesticide formulation. pH: If the degradation reactions in soil are acid or base catalysed, then pH definitely influences the rate of degradation. The negatively charged soil surface results in a higher concentration of hydrogen-ion near the soil surface than the bulk solution. Further, if the pH reduces, the negative charge on the soil surface decreases and is more capable of adsorbing the anions. For example, sulphonylureas persist in alkaline chalky subsoils as these get degraded by acid-catalysed hydrolysis reactions. However, oxime-carbamates persist in acid subsoils since these get degraded by base-catalysed reactions (Van Raij and Peech, 1972). Depth: With depth, microbial activity and organic matter in soil decreases, possibly exposes clay but resulting in weaker adsorption. Due to a decrease in microbial population and organic matter in the soil, the degradation rate of pesticides decreases. However, special processes may take place with depth e.g. by ferrous ion catalysed anaerobic reduction reaction, oxamyl is exceptionally degraded in acidic sandy subsoils (Leistra, 1986). The soil adsorption coefficient (Kd) also positively correlates with herbicide depth movement. The dynamics (soil movement, adsorption and dissipation) of herbicides namely; simazine, diuron, pendimethalin, oxyfluorfen and flumioxazin was studied in the sandy loam soil in Casablanca Valley, Chile. The movement in soil was 120 cm in simazine, 90 cm in diuron and 30 cm in flumioxazin. Oxyfluorfen and pendimethalin were found in top 5 cm layer (Kogan et al., 2007), and 2, 4-Dichlorophenoxyacetic acid (2, 4-D) being widely used against the broad leaf weeds has posed a risk to ground water. The 2, 4-D and its metabolite, 2, 4-Dichlorophenol (2, 4-DCP) gets adsorbed, desorbed, degraded and displaced in soils having organic matter, iron, aluminium oxide and clay. The distribution coefficients for 2, 4-DCP sorption were linear and more than 2, 4-D. The increased preferential flow due to porous soil and high soil clay content has further increased risk to groundwater contamination by reducing the soil’s buffering capacity (Prado et al., 2016). Soil texture: According to Brown (2012), soil texture affects the movement of water passing through it, thereby affecting the movement of pesticides dissolved in water. In coarser soils, percolating water moves faster, and the chances of adsorption of pesticide to the soil particles is less. Soils with clayey texture have more surface area onto which more pesticides can get adsorbed and hold water for longer period. Miscellaneous factors Factors like weather, climate and irrigation practices affect the pesticide degradation and leaching. If the soil temperature is warmer, there is an increase in degradation and if cooler then degradation gets retarded (Anonymous, 2019). IF watering is done in large quantity to the field it favours pesticides and fertilizers to leach down and get distributed deep into the soil profile and finally reach the groundwater. According to a study on pesticide accumulation in soil that has been done in Mezquital Valley (Central Mexico) on maize crop, the crop treated with atrazine (weedicide) for over 20 years resulted into two metabolites in the soil namely; hydroxyatrazine and deethylatrazine. Hydroxyatrazine was detected after first and third irrigation in the soil to a concentration of 0.08 mg kg-1, while deethylatrazine got percolated down with second irrigation and was detected in percolation water only (0.03 mg L-1). The mobility potential of 32 pesticides viz., carbaryl, carbofuran, chlorpyriphos, dimethoate, quinalphos, imidacloprid, thiamethoxam, thiodicarb, quinclorac etc. on surface and groundwater was assessed using pesticide risk indicators in the paddy fields of Mahaweli river basin in Sri Lanka. Among all the pesticides, carbofuran, thiamethoxam, and quinclorac were the most leachable pesticides. The most mobile pesticides in the soil are carbofuran and quinclorac as a result of runoff, while thiamethoxam and novaluron are by means of erosion (Aravinna et al., 2017). The studies on mobility of carbofuran and diuron in forty-three different soil surfaces (dry and wet zones) of Sri Lanka revealed that both pesticides have high adsorption coefficient (Kd). Sorption for both was two times higher in dry zone than in wet zone (Liyanage et al., 2006). Degradation Organic pesticides are major sources of pesticide property, environmental conditions, etc. (Rao et al., 1983). As far as the microbial transformation of various pesticides is concerned, it is expected that the chemical compounds can be present in the soil, water and plant, and toxicity may be more or less than the soil pollution in agricultural lands. Most of these pesticides are persistent and tend to bio accumulate in humans upon consumption of contaminated plants (El-Saeid et al., 2022). Pesticide degradation is a fundamental process for pesticide residue attenuation (Guo et al., 2000) and is governed by biotic and abiotic factors, viz., microbial interactions, soil composition, pesticide property, environmental conditions, etc. (Rao et al., 1983). As far as the microbial transformation of various pesticides is concerned, it is expected that the chemical compounds can be present in the soil, water and plant, and toxicity may be more or less than the parent compound (Chowdhury et al., 2008). Biodegradation/ microbial degradation Biodegradation/microbial degradation is a natural instinct of microbes, a strategy for survival. Efficient and environmentally friendly microbial degradation has been considered as the most promising remediation method (Pang et al., 2022). The microbiota (especially bacteria) in the soil flourish in the presence of available pesticides (Guillen-Garces et al., 2007), which act as electron donors, sources of carbon and other nutrients, thereby causing pesticide degradation (Qiu et al., 2007). Some microbes are modified to degrade pesticides rapidly and thus microbes with such capabilities can be utilized for removing contaminants from the actual site of application. Pesticide biodegradation is influenced by the factors such as soil organic matter, moisture content, soil texture, aeration, temperature, pH etc. and is highest in well aerated, moist, warm soil with a neutral pH. The soil profile with high organic matter in the surface soil shows a higher rate of microbial degradation whereas the rate of degradation is inversely related with the depth of soil because conditions of temperature, aeration, and moisture are not favorable for activity of soil microbes. To achieve precise bioremediation, it requires an understanding of the genetics and physiology of the desired microbe. The reaction rate of degradation reactions aided by microorganisms is enzyme conciliated, and it doubles for each 10°C rise in temperature with temperatures ranging between 10 and 45°C. Enzymatic activity is colossally reduced above and below these temperatures. The gene encoding the enzymes for degradation has been identified for various pesticides and can be used for a super strain development to accomplish the desired results (Singh, 2007). In the absence of a microbial system for pesticide degradation, it leads to pesticide persistence in soil which is also affected by other factors like pesticide distribution pattern between soil and foliage, temperature, soil characteristics such as composition, soil pH, water pH etc. (Singh and Thakur, 2006). In recent years, a number of microbial floras viz. bacteria, actinomycetes, fungi etc., have been isolated and screened for degradation of pesticides. The soil-inhabiting bacterial genera namely; Bacillus, Arthrobacter, Pseudomonas and Micrococcus, constitute the majority group involved in organochlorine degradation (Langlois et al., 1970). Rana et al. (2015) isolated bacteria species namely; Pseudomonas putida strain IMBL 5.2 and Bacillus aeromonas strain IMBL 4.1 for thiamethoxam degradation which resulted in 38.23 and 45.28 per cent thiamethoxam (50 μg mL-1) reduction, respectively, in 15 days and proved to be potential thiamethoxam degrading species. Pseudomonas sp. has also been reported as dieldrin degrading bacteria (Matsumura et al., 1968). Endosulfan degradation by Pseudomonas aeruginosa (88.5%), Stenotrophomonas maltophilia (85.5%), Acinetobacter lowffii (80.2%), Bacillus atrophaeus (64.4%) and Citrobacter amolonaticus (56.7%) was reported by Ozdal et al. (2016). For detection of endosulfan degradation, Kafilzadeh et al. (2015) isolated bacteria from water samples and sediments from agricultural land. The bacteria oxidised the endosulfan to carbon dioxide and water, which acted as a source of carbon and energy, thereby supplementing their growth and reproduction. Several bacteria competent of degrading aldicarb, carbofuran, carbaryl or baygon (Larkin and Day, 1986), endosulfan (Hussain et al., 2007), monocrotophos (Subhas and Singh, 2003) etc. have been identified. The ‘Esd’ gene from Arthrobacter sp. encodes for an enzyme (monooxygenase) that degrades endosulfan α and ß (Sutherland et al., 2002; Weir et al., 2006). The ‘lin’ gene has been characterized in Sphingomonads namely; Spingobium indicum, Spingobium francense and Spingobium japonicum, responsible for uptake, pesticide metabolism, and successful degradation of hexachlorocyclohexane (HCH) (Pal et al., 2005). The enzyme HCH dehydrochlorinase encoded by ‘lin’ gene mediates dehydrochlorination of HCH (Lal et al., 2006). Thus, for the development of bioremediation technology for pesticides like HCH, the diversity, distribution and genetics of lin gene should be deciphered Also, the bacterial degradation of α-endosulfan and ß-endosulfan by Staphylococcus, Bacillus circulans-I and B.circulans II has also been reported by Kumar and Philip (2006). Some other enzymes responsible for biodegradation of pesticides are enlisted in Table 1. The fungal species are also potent candidates for pesticide degradation. Kamaei et al. (2011) reported efficient degradation of endosulfan and endosulfan sulphate by white rot fungus, Trametes hirsuta. Trichderma harzianum and Rhizopus nodosus isolated from pesticide contaminated soil degraded 70 to 80 per cent of chlorpyriphos and ethion within 21 days (Harish et al., 2013). For the carbofuran tolerance test, 319 actinomycetes were selected by Jayabarath et al. (2010) from Maharashtra, and they observed that strains of Streptoverticillium album, Micromonospora chalcea, Streptomyces alanosinicus, Nocardia farcinia, Nocardia vaccini, Streptomyces atratus and Nocardia amarae were potent enough to grow and degrade pesticides. Lin et al. (2011) isolated cypermethrin degrading strain HU-S-01 of Streptomyces parvulus from waste water sludge as a potential biological agent for decontamination of cypermethrin contaminated crop, soil or water. Some of the pesticide degrading microbial organisms have been enlisted in the Table 2. In addition to bacteria, fungal cultures are also effective tools in the bioremediation of pesticides. Fungal hyphae secrete extracellular enzymes that can penetrate deeper into the soil matrix with a larger surface area than bacterial isolates (Shi et al. 2018; Guo et al. 2019). Bisht et al. (2019) screened 20 fungal isolates for their ability to degrade endosulfan and chlorpyriphos. Both α- and β-endosulfan isomers were completely degraded by Trametes hirsuta and Cladosporium cladosporioides resulted in maximum degradation of chlorpyrifos. Chemical degradation Chemical degradation reaction takes place in the presence of water, oxygen or other soil chemicals, and under extremes of soil pH and microbial activity. However, such conditions may be in favor of chemical degradation at a faster rate (Kerle et al., 2007). The different ways of pesticide degradation include photolysis, reduction, oxidation, hydrolysis, decarboxylation, dehydrogenation, condensation, dehalogenation etc. Photolysis can be enhanced by adding humic materials or other additives like riboflavin, rose bengal, methylene blue, etc. that act as photosensitizers (Miller et al., 1987). Hydrolysis is another chemical process involved in pesticide degradation. Wei et al. (2001) considered it a primary transformation pathway for carbamates in the environment. Photodegradation Photodegradation has become an important index in evaluating the environmental safety of pesticides. It significantly influences the pesticide fate on crop surface (residue, efficacy and toxicity), in the atmosphere and water (Hong and Zeng, 2002). Almost all chemical pesticides are photodegradable to some extent, influenced by sunlight intensity, exposure period and pesticide properties. Foliage and soil surface applied pesticides are more prone to photodegradation than soil incorporated. Pesticide degradation is at a faster rate inside plastic cladded greenhouses than glasshouses as glass reflects back much of the ultraviolet rays that are having the potential to degrade pesticides (Kerle et al., 2007). Mechanism of microbial degradation Enzymes are of paramount importance for xenobiotic degradation in the soil and play an important role inpesticide degradation. After getting into the microbes, these pesticides undergo some biochemical and physiological changes aided by enzymatic reactions, thereby getting transformed into some less toxic or non- toxic compounds (Parte et al., 2017b; Tang, 2018). Kim et al. (2004) proposed the metabolic pathway for degradation of carbofuran by bacteria like Arthrobacter sp and Sphingomonas sp. The first product of carbofuran degradation is carbofuran phenol followed by the formation of 2-hydroxy-3-(3-methylpropan-2-ol) phenol. The bacterial microorganisms are known to combat secondary pollution as they help transform toxic organic molecules into the non-toxic ones (Prabha et al., 2017). Some microbes have enzymes that enable them to degrade the pesticides and convert the organic compounds to inorganic ones by a process called mineralization. These microbes utilize these pesticides as a source of their nutrients. Another process for degradation of pesticides involves co-metabolism in which pesticides could be degraded by adding some organic matter like exo-genous or iso-biomass as the primary energy source (Zhang et al., 2010). Risk to groundwater contamination Groundwater is a major source of fresh water for the global population and is used for domestic, agricultural and industrial uses. Approximately one third of the global population depends on groundwater for drinking water (International Association of Hyrogeologists 2020). Pesticide use has undoubtedly resulted in improved crop production by preventing the damage by the pests but has increased the risk of groundwater contamination, especially in urban areas. The quality of drinking water has deteriorated in recent times. The reason is, the increasing population pressure, unplanned growth of cities, mixed land use patterns, poor sewage facilities, unplanned or illegal disposal of wastewater from industries and households. Shallow aquifers have been polluted to a greater extent due to industrial and domestic effluents. In India, water pollution is a major concern, as the groundwater reserves and about 70 per cent of its surface water resources are already contaminated by organic, inorganic and biological pollutants (Rao and Mamatha, 2004). Urban areas in India have accessibility to only groundwater, so the quality and quantity of groundwater are equally important. With the increasing awareness regarding groundwater contamination, efforts have been made to prevent or reduce groundwater pollution (Kalinski et al., 1994). The contaminated groundwater limits its use in agricultural areas as a source of crop irrigation and thus has become a global concern. Several problems like human diseases, soil fertility degradation and reduced farmers’ income may result from the use of contaminated groundwater (organic and inorganic contaminants) for irrigation. The impacts of this contaminated groundwater are more pronounced in intense agricultural areas where sufficient water is not enough to irrigate crops (Komnitsas et al., 2015). Sources of groundwater pollution Groundwater contamination is a global problem that has a significant impact on human health and ecological services (Li et al., 2021). Risk to groundwater refers to, “the probability that groundwater will suffer unacceptable contamination due to human activities”. The concentration and type of groundwater contaminant in the solution depends upon the point source of groundwater, rate of groundwater movement, and surface and subsurface environment (Prasad et al., 1999). It has become important, especially in agricultural areas, to recognize groundwater pollutants and evaluate the risk involved in pesticide use. Pesticides and nitrates are dominant non-point contamination sources in agricultural fields especially for the shallow alluvial aquifers. Factors for the vulnerability of alluvial aquifers include high permeability of alluvial deposits, shallow water table, interconnections between surface water and agricultural related land uses, and sea water intrusion due to over-pumping of groundwater for irrigation (Guler et al., 2012). Rainwater is relatively free of minerals till it comes in contact with various constituents present in the soil, where pesticides, minerals and salts get dissolved in it, which are carried into the solution as water moves through the aquifer. Various ion concentration, viz., cations and anions, is dependent on mineral solubility, minerals present in the solution, the time duration for which water is in contact with the rocks and the amount of dissolved CO2 present in the water (Viessman et al., 1989). Various factors affect the chemical alteration of the ground water: solid phase interaction, polluted river water seepage, the residence time of ground water, saline water getting mixed with groundwater, and anthropogenic impacts (Umar and Absar, 2003). Various pesticides have been reported as groundwater contaminants. Atrazine has been found to bind easily to aliphatic and aromatic compounds that have posed a risk to groundwater contamination (Dutta et al., 2015). Mittal et al. (2014) reported the enhanced leaching capacity of pesticides through silty textured soil into groundwater in the Malwa region of Punjab. For this reason, it has been described as India’s ‘cancer capital’, consuming almost 75 per cent of total pesticide consumption in Punjab, leading to poor groundwater quality. Jayashree and Vasudevan (2007) reported DDT, HCH and endosulfan residues from groundwater of Thiruvallur district of Tamil Nadu, India. Residue of endosulfan sulphate (metabolite of endosulfan) was detected in a range of 15.9 μg L-1 in the bore wells, γ-HCH was found to be as high as 9.8 μg L-1 in open wells and pp-DDT (DDT isomer) was in the range of 14.3 μg L-1. For land-use planning and management, and protection of groundwater, risk assessment is an imperative tool. Though detection and control of groundwater contamination is a tough task than dealing with surface water as they may persist for many long years or even centuries (Todd, 1980) Even then there are some major methods involved in risk assessment of ground water pollution namely; index method, process-based methods, statistical methods and fuzzy comprehensive evaluation method. There are also other methods for pollution risk evaluation based on measuring solubility, degradation and sorption. Gustafson (1989) developed a leaching risk index based on groundwater contamination. In addition to giving an index/score, it predicts the probability of the chemical being present in groundwater (Worrall et al., 1998). Factors affecting groundwater pollution Pesticide flux in soil is one of the important factors affecting groundwater contamination. Chemical pesticides rapidly pass to groundwater when they cross the unsaturated soil zone. This movement is controlled by water. The hydraulic gradient and hydraulic conductivity of the soil, govern the rate with which water flows through the soil profile. The size, shape, and interrelation of the soil pores regulate the conductivity, which varies according to the soil type. Gas phase also controls the water flow paths in the unsaturated zone above the water-table (Rao et al., 1988). The pesticide properties like soil dissipation half-life and soil organic-carbon partition coefficient influence pesticide leaching in the soil. Also, the soil properties like structure, clay content, organic matter, and soil hydrological processes influence the magnitude and rate of pesticide transportation in soil. Wauchope et al. (2001) correlated pesticide mobility and its leaching risk into groundwater, especially for hydrophobic ones with weak sorption on the soil matrix. They observed that the ratio of adsorbed pesticide to the solution-phase pesticide got normalized with respect to organic matter content in the soil. However, Elliott et al. (2000) observed pesticides with adsorption constant (Koc) 1000 got transported into groundwater shortly after application due to heavy rainfall. In the tropical regions, animal galleries viz., termite galleries are the preferential routes of flow under rainstorms (McGarry et al., 2000). In addition to these, some other factors which affect pesticide pollution of groundwater are (Anonymous, 2017): adjuvants (solvents or diluents, emulsifiers, wetting agents, adhesives, extenders, preservatives and buffers) that are mixed with the active ingredient; products formed during chemical, photochemical or microbial degradation of the active ingredient; pesticide mobility in soil, both horizontally and vertically in the soil structure, e.g. residual herbicides are designed such that they bind directly to the soil; contaminants in the active ingredient; half-life of pesticidesPesticides that take longer time to break down have higher stability and persistence; pesticide application rate and solubility in water; microbial activity and soil temperature etc. Top Conclusions Pesticide retention by soil prevents its short-term admittance to the surface and groundwater. Still, non degraded pesticides and their metabolites result in an interminable risk to the environment and human health. So, an adequate understanding of these processes, i.e. retention, mobility and degradation of pesticides is essential Degradation of pesticides by a complex of methods provides an important tool for decontamination of areas affected by the overuse of pesticides. Biodegradation is an environment-friendly approach for reducing the risk associated with use of hazardous chemicals. Hence, the scrutinization of highly efficient microbial strains for pesticide degradation to reduce environmental pollution is the need of the hour. |
Top Figures Fig. 1.: Trend of pesticide (technical grade) consumption in India
| 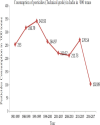 | |
| Fig. 2.: A flow chart representing the mobilization of pesticide in the environment
| 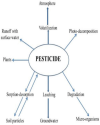 | |
| Fig. 3.: Environmental factors affecting the pesticide degradation
| 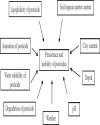 | |
| Fig. 4.: Degradation pathway of neonicotinoid pesticides and their metabolites formed in the soil
| 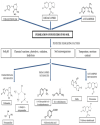 | |
|
Tables Table 1.: Enzymes responsible for bioremediation of pesticides (credit: Ortiz-Hernández et al., 2013)
| Enzyme | Organism | Pesticide | Oxidoreductases (Gox) | Pseudomonas sp. LBr Agrobacterium strain T10 | Glyphosate | Monooxygenases: ESd | Mycobacterium sp. | Endosulphan and Endosulphate | Ese | Anthrobacter sp. | Endosulphan, Aldrin, Malathion, DDDT and | Cyp1A1/1a2 | Rats | Endosulphate Atrazine, Norflurazon and Isoproturon | Cyp76B1 | Helianthus tuberosus | Linuron, Chlortoluron and Isoproturon | P450 | Pseudomonas putida | Hexachlorobenzene and Pentachlorobenzene | Dioxygenases (TOD) | Pseudomonas putida | Herbicides Trifluralin phosphotriester | E3 | Lucilia cuprina | Synthetic pyrethroids and insecticides | Phosphotriesterases: OPH/OpdA | Agrobacterium radiobacter, Agrobacterium radiobacter, Pseudomonas diminuta, Flavobacterium sp. | Insecticides phosphotriester | Haloalkane | Flavobacterium sp. Sphingobium sp., Shingomonas sp. | Hexachlorocyclohexane (β and δ isomers) | Dehalogenases: LinB | | | AtzA | Pseudomonas sp. | Herbicides chloro-s-trazina | TrzN | Nocardioides sp | Herbicides chloro-s-trazina | LinA | Sphingobium sp., Shingomonas sp. | Hexachlorocyclohexane (γ isomers) | TfdA | Ralstonia eutropha | 2,4 - dichlorophenoxyacetic acid and pyridyl-oxyacetic | DMO | Pseudomonas maltophilia | Dicamba |
| | Table 2.: Enlisting some microorganisms involved in pesticide degradation
| Type of microorganism | Pesticide degraded | Scientific name | References | Bacteria | Fenitrothion | Burkholderia sp., | Hong et al., 2007 | Isoproturon | Sphingomonas sp. | Bending and Rodríguez-Cruz, 2007 | Methyl parathion | Ochrobactrum sp. | Qiu et al., 2007 | Diuron | Micrococcus sp. | Sharma et al., 2010. | Methyl parathion | Sphingobium sp. | Yuanfan et al., 2010. | Endosulphan | Pseudomonas sp. | Verma et al., 2014 | Chlorpyrifos | Bacillus sp. | Verma et al., 2014, Upadhyay and Dutt, 2017 | Glyphosate | Flavobacterium | Upadhyay and Dutt, 2017 | Dichlorovos | Pseudomonas stutzeri | Parte et al., 2017a | Fungus | Endosulfan | Mortierella sp strain W8 and Cm1-45 | Shimizu, 2002 | Pirmicarb | Trichoderma viridae, Trichoderma harzianum | Eapen et al., 2007 | β-Cypermethrin | Aspergillus niger | Deng et al., 2015 | Actinomycetes | Carbofuran | Streptomyces allanosinicus | Wu et al., 2004 | Chlorpyrifos | Streptomyces sp strain SJRO-06 and SJRO-10 | Sunitha and Onkarappa, 2018 |
| |
|