|
|
|
Field Resistance to Cyantraniliprole in Plutella xylostella Elakkiya K1, Murugan M1,*, Krishnamaoorthy S V1, Senthil N2, Vijayalakshmi D3 1Department of Agricultural Entomology, Tamil Nadu Agricultural University, Coimbatore641003, Tamil Nadu, India 2Department of Plant Molecular Biology and Biotechnology, Tamil Nadu Agricultural University, Coimbatore641003, Tamil Nadu, India 3Department of Crop Physiology, Tamil Nadu Agricultural University, Coimbatore641003, Tamil Nadu, India *Email: muruganmarimuthu@tnau.ac.in (corresponding author): ORCID ID 0000-0002-7485-1153
Online Published on 04 January, 2024. Abstract Cyantraniliprole belongs to new chemical class of diamides and used Plutella xylostella one of the highly devastating lepidopteran insect pests of cole crops has been found to develop resistance to cyantraniliprole worldwide including India. However their mechanism of resistance is not examined in Indian scenario. Hence a survey was conducted to analyse the current resistance status of field population of P. xylostella to cyantraniliprole. The maximum lethal concentration expressed by field population in Tamil Nadu was 19.81 ppm which had higher ratio (2828.57) of resistance to that of susceptible population. The enzyme analysis revealed the over expression of GST up to at 24 hr after exposure (HAE) and MFO up to 48 hr upon exposure. Further the synergistic study stated that PBO and DEM could increase the toxicity of cyantraniliprole to some extent. Top Keywords Cyantraniliprole, MFO, GST, CarE, Plutella xylostella, LC50, synergist, Cole crops, Resistance, Toxicity, Ratio of resistance, Enzyme. Top | India ranks second in cabbage and cauliflower production worldwide and these are affected by number of pests. The diamondback moth (DBM) Plutella xylostella, (Lepidoptera : Plutellidae) causes 50-80% yield loss (Uthamasamy et al., 2011). The larvae of DBM is oligophagous and are destructive feeders of brassica plants. The DBM challenge is often worst in tropics and subtropics, where round the year cultivation of brassica crops is in vogue (Grzywacz et al., 2010). Farmers use intensive applications of insecticides to check DBM menace, even about 14-16 sprays (Biradar, 2020), often accounting for one spray for each week (Abhijith et al., 2019). However due to selection pressure (Hawkins et al., 2019), DBM had resisted several insecticides, by evolving resistance mechanisms. In addition, since DBM has a shorter life cycle with 25 generations annually, a stronger selection pressure is getting experienced. The high fecundity rates in DBM also support evolution of resistance (Wright, 2004). To date a variety of field-applied insecticides have become ineffective (Kim et al., 2011). Diamides with two distinct subclasses viz., phthalic (flubendiamide) and anthranilic (chlorantraniliprole and cyantraniliprole) are one among the newer type of insecticides which inhibit further muscular contraction and results in paralysis and finally death among targeted insects (Sattelle et al., 2008). Lepidopterans are the primary targets of diamides but these failed to manage DBM (Troczka et al., 2012) and later on, diamide resistance was noticed in several other lepidopteran insects (Yao et al., 2017; Huang et al., 2021). In India, flubendiamide, chlorantraniliprole and cyantraniliprole are the three commercial formulations of diamides approved for use since 2007. Diamides are the major used insecticides in the fields of cabbage and cauliflower in India (Biradar, 2020; Anjali et al., 2018). Field populations of DBM were found to exhibit resistance to these (Shanmugapriya et al., 2019). Several reports worldwide emanated adjudging insecticide resistance mechanisms in DBM (Jiang et al., 2015; Banazeer et al., 2021). But, in India the mechanism mediating such resistance against diamides in DBM is unexplored, and hence the present study with a survey on the susceptibility of P. xylostella to cyantraniliprole in the major cabbage and cauliflower growing zones of Tamil Nadu. This study was done also to identify the enzymatic mechanisms that rule the cyantraniliprole resistance. |
Top Materials and Methods The field population of P. xylostella were collected from cauliflower in Coimbatore in 2020 and was maintained in the laboratory to develop the susceptible population. The laboratory reared larvae were fed with insecticide free leaves of cauliflower (Brassica oleracea var. botrytis, cv. Snow White). The larvae upon pupation, were collected separately and placed safely in the fine meshed cage (45x45x45 cm). The emerged adults were supplemented with 10% sugar solution. The adults were allowed to lay eggs on the young (4-5 days old) mustard (Brassica nigra, hybrid: Pioneer 45s46) seedlings and left uninterrupted until egg larval hatch. Then, the neonates were transferred in to contamination- free cauliflower leaves. The whole rearing was performed under a controlled condition (27±2°C; 50-70% RH; 16:8 L:D). Field populations of P. xylostella were collected for resistance assessment in the major cole crops cultivating areas of Tamil Nadu (covering three agroclimatic zones of seven districts) during 2021-2022. Field-collected populations were kept in cages apart from susceptible cultures under controlled environment, as explained for susceptible cultures. |
Leaf dip bioassay was conducted based on IRAC (Insecticide Resistance Action Committee) susceptibility test method no: 018 using the commercially available cyantraniliprole. To conduct bioassay, cauliflower leaf discs (4.5 cm) were dipped in different concentrations of insecticide solutions and finally added with Triton X-100 (1 g/ ℓ) as a surfactant. The control was prepared by treating leaf discs with distilled water containing Triton X-100 (1 g/ ℓ) and each treatment including control was replicated thrice. About 10 uniform sized late second instar larvae were selected, starved and released in each replication. Then the larval mortality was assessed after 72 hr. The impact of synthetic synergists (PBO, DEM and TPP) and non-edible oils (rosemary oil (RO) and thyme oil (TY)) on the toxicity of cyantraniliprole to two field populations and lab maintained susceptible population were also assessed using the common leaf disc bioassay as described above. The synergist (100 mg/ ℓ) was then added to each concentration of cyantraniliprole as they exhibited no toxicity effects when tested alone and the toxicity was assayed as mentioned in bioassay described above. |
The activity of detoxifying enzymes such as mixed function oxidase (MFO), glutathione S-transferase (GST) and carboxyl esterase (CarE) were assessed in both susceptible and the most resistant populations (NIL and KRI) of P. xylostella at different hours after exposure (HAE) (viz., 3, 24 and 48 HAE) to cyantraniliprole at sublethal (LC25) quantity. Alive, healthy, late second instar larvae after insecticide exposure were weighed and homogenized under ice cold conditions using pestle and mortar. The following buffers : 0.1M Tris buffer, 10 mM glutathione reduced, pH 8.0) for GST; 50 mM ice cold tris buffer, 1.15% KCl, and 1.0 mM EDTA, pH 7.5 for MFO; and 0.1M phosphate buffer, pH 7.5, containing 0.1% Triton X-100 for CarE were used. Then the extracted contents were centrifuged at 10000 rpm, at 4°C for 12 min and the supernatant was stored at -20°C to quantify respective enzymes. For protein assay bovine serum albumin (BSA) was used as a protein standard, the Bradford method was used to quantify the total protein content (Bradford, 1976). Using p-nitroanisole as the substrate, MFO was measured in accordance with Yasoob et al. (2018) with a few changes. One 100 μl of the enzyme source was mixed with 1 ml of 50 Mm p-nitroanisole (in ethanol) and 200 μl of 10.0 mM NADPH and incubated in darkness for 3 min at room temperature. Using BioSpectrometer®, the absorbance of the end product, p-nitrophenol, was measured at 405 nm for 3 min. at the interval of 30 sec. Using the extinction coefficient of 3.32 mM-1cm-1, the activity of MFO was estimated (Sharma, 2017). Quantification of GST was done according to Kao et al. (1989) using CDNB (0.1M) as a substrate. 0.1M tris buffer was added to one hundred μl of enzyme source followed by adding 0.1 M CDNB (20 μl). Then enzyme activity was quantified using spectrophotometer (BioSpectrometer®) at the wavelength of 340 nm for 3 min at 30 sec. interval. The extinction coefficient of CDNB - 9.6 mM-1 cm-1 was used to estimate the activity of GST. Quantification of CarE was carried out using the substrate 1-naphthyl acetate as described by Gong et al. (2013) with slight changes. Chilled phosphate buffer 0.04 M (450 μl) and 1-naphthyl acetate 0.3mM (1.80 ml) was added to one hundred μl of enzyme source and incubated at room temperature for 30 min. Two parts of fast blue RR salt and five parts of sodium dodecyl sulphate was added to the reaction mixture and absorbance was taken after 15 min at wavelength of 600nm. To calculate the specific activity of CarE, the extinction coefficient of 1-naphthyl acetate (2.22 mM-1cm-1) was used (He, 2003). |
The obtained control mortality was >10 % and was corrected using Abbott’s formula (Abbott, 1925). Further the mortality data was subjected to Probit analysis to obtain LC50, LC95, their respective fiducial limits, chi square (χ2) and slope. Resistance ratio (RR) was calculated by dividing the LC50 values of field population with the LC50 of susceptible population. The insect population was categorised as susceptible, decreased susceptible, low resistance, moderate resistance, high resistance and very high resistance according the values of resistance ratio RR (<3.0, 3.1-5, 5.1-10.0, 10.1-40.0 40.1-160.0 and >160.0, respectively). Likewise the synergist ratio (SR) was calculated using LC50 values of field population with synergist divided by LC50 values alone. With IBM SPSS® Statistics 22.0, the data relevant to the measurement of various enzyme activities were analysed using one-way ANOVA, (p <0.05), followed by the Tukey’s test (Tukey, 1977) for mean comparison. |
Top Results and Discussion The concentration-mortality responses of the P. xylostella to cyantraniliprole were extremely a good fit by the probit model. The lab maintained population though not a true susceptible population exhibited significantly low LC50 (1.50 ppm) to cyantraniliprole at its 27th generation than any other field population. Among the field collected P. xylostella population, NIL that belonged to hilly and high altitude zone of TN exhibited significantly highest LC50 (19.81 ppm) than any other field population (Table 1). This was followed by KRI (9.51 ppm) and CBE population (8.53 ppm) belonging to North Western zone and Western zone respectively however, CBE population exhibit faster rate of resistance development than other field population as their fiducial limits were on par with the limits of NIL population while the rate of resistance development in other field populations (KRI, DIN, DHA, TIR and THE) were similar. When calculated using LC50 of susceptible population engaged in the present study, the RR was found very low (3.4413.20) calculated for cyantraniliprole among all field population studied and were grouped in to decreased susceptible to moderate resistance categories, however at the same time on comparison to that of the susceptible DBM population used in Shanmugapriya et al. (2019) utilized in earlier work from same TNAU Entomology laboratory provided RR adjudged as extremely high (RR=950-2829) and pointed that the these populations studied were categorised in to very high resistance group (Table 1). |
High resistance (RR=2024) to cyantraniliprole was also reported in the field population of P. xylostella from Brazil by Ribeiro et al., (2017) and from many parts of the world (Dunn et al., 2022; Pasupathi et al., 2022). There were also reports of cross resistance in P. xylostella and other insects to diamide insecticides as they possess similar mode of action (Bolzan et al., 2019; Liu et al., 2015). Thus, field population studied in the present study too found to have a high ratio of resistance to cyantraniliprole though the fields sprayed with other diamide like flubendiamide and chlorantraniliprole (Anjali et al., 2018) suggesting the occurrence of cross resistance to cyantraniliprole. Thus, the present findings are similar with those of Liu et al. (2015), who encountered the occurrence of cross resistance in P. xylostella within diamide insecticide group. |
In the resistance populations (NIL, KRI) of P. xylostella, the activity of MFO was found to increase at steady rates significantly (P < 0.05) from 3 to 48 HAE to cyantraniliprole (Fig. 1). The activity of MFO in laboratory maintained susceptible was found significantly at decreased level at 24 HAE, however there was an increase in the level noticed at 48 HAE, indicating the involvement of MFO to some extent. In addition, the activity of GST was found to increase significantly up to 24 HAE in both laboratory maintained susceptible and resistance populations (NIL and KRI), with maximum level (3.48 (μmol/min/mg of protein) registered in NIL at 24 HAE. Thus, our results depicted the association of MFO and GST involvement to some extent in resistance development aspect of P. xylostella to cyantraniliprole. While the activity of CarE was found to be either increased or decreased significantly irrespective of the time of insecticide exposure in all the tested insect populations. Further, lower activities of CarE in resistant populations (NIL, KRI) than laboratory reared susceptible population were observed, which had pointed out the limited role of CarE activity in the resistance development contribution prospects of P. xylostella against cyantraniliprole. |
Insecticide resistance in insects follow several known and well established routes besides yet to be explained unknown strategies too. However, broadly studied mechanisms are based on either increase or decrease in expression detoxifying enzymes or by mutations happening in the target sites on their combinations (Feyereisen, 1995; Ffrench-Constant, 1999). According to Arthropod Pesticide Resistance Database (APRD), P. xylostella, a widely studied insect around the globe is found to resist about 101 insecticidal compounds including diamides with various resistance mechanisms viz., decreased cuticular penetration, increased activity of detoxifying enzymes and changes in target-sites that crop up through genetical routes such as point mutations, amino acid substitutions, and reduced or no binding to target-sites (Banazeer et al., 2021). Thus, in line to previous reports, in current study, it was observed that the resistant population of P. xylostella was tracked to express higher activity of MFO (3.03 fold) and GST (2.25 fold) at 48 and 24 HAE, respectively than susceptible population. The higher activity of GST and MFO was found in resistance than susceptible populations when exposed to chlorantraniliprole (Kang et al., 2017), a similar chemistry to cyantraniliprole. Likewise, Hu et al. (2014) suggested the stronger involvement of GST mediated resistance to chlorantraniliprole, while MFO and CarE were involved to some extent only. Since cross resistance reports between chlorantraniliprole and cyantraniliprole among DBM were previously documented (Liu et al., 2015; Zhang et al., 2016;), exposure to any one of them itself is enough it seems for the resistance expression against them and it may be construed that the mechanism(s) of resistance might be more or less similar between these two insecticide compounds. Anyhow, in yet another insect whitefly Bemisia tabaci (Hemiptera: Aleyrodidae), with a different mode of feeding, Zang et al. (2022) reported the involvement of cytochrome P450 (CYP) genes in the resistance against cyantraniliprole. But, there are reports of target site mutations to diamides including cyantraniliprole (Dunn et al., 2022). |
The effect of synthetic synergists viz., PBO, DEM and TPP and non-edible essential oils (RO and TY) were shown in Table 2. When P. xylostella was treated along with synergist, the LC50 of cyantraniliprole was found to decrease in all the populations viz, laboratory maintained susceptible (1.33 to 0.29 ppm), NIL (16.81-6.91 ppm) and KRI (8.99-2.85 ppm). The SR of PBO were found to be 2.08, 2.87 and 1.99 and for DEM, the SR registered were 5.17, 2.76 and 3.34, in susceptible, NIL and KRI populations, respectively. But, the SR registered for remaining synergists, TPP, TY or RO (1.06-1.85) were very lower in all the three population studied. A similar reduction of LC50to cyantraniliprole by use of PBO and DEM was previously also documented (Liu et al., 2015). But, synergists like TPP, TY or RO were not effectively participated in breaking the resistance of P. xylostella to cyantraniliprole. But still there are several research reports that these synergists increases the toxicities of insecticides and acaricides (Wu et al., 2007; Zhang et al., 2014; Tak et al., 2017; Raghavendra et al., 2017). |
Thus, the results of present study provides documentation of the build up diamide resistance in field population of P. xylostella in cole crops growing tracts of Tamil Nadu. |
Top Figure Fig. 1.: Activities of MFO, GST and CarE detoxifying enzymes in the laboratory maintained susceptible and two most resistant field population of P. xylostella hours after exposure to cyantraniliprole
| 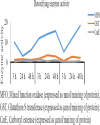 | |
|
Tables Table 1.: Toxicity of cyantraniliprole to field population of P. xylostella from different agroclimatic zones of Tamil Nadu, India
| Agro climatic zones | Location | Population | n | Slope± E | χ2(df) | LC50 (ppm) (95% FL) | LC95 (ppm) (95% FL) | RR1 | RR2 | Western zone | Susceptible | – | 180 | 1.31±0.33 | 2.22(3) | 1.50 (0.95-2.09) | 14.38 (6.60-127.67) | – | – | Coimbatore | CBE | 180 | 1.47±0.49 | 2.58(3) | 8.53 (6.06-25.12) | 63.79 (22.92-7894.58) | 5.68 | 1218.57 | Theni | THE | 180 | 1.22±0.34 | 0.12(3) | 5.20 (2.23-7.83) | 58.51 (28.39 -570.10) | 3.44 | 742.86 | | Tiruppur | TIR | 180 | 0.90± 0.25 | 1.09(3) | 6.65 (3.26-11.79) | 175.65 (53.84-8430.54) | 4.43 | 950.00 | | Dindigul | DIN | 180 | 2.37±0.41 | 0.69(3) | 6.83 (5.32-8.46) | 23.74 (16.97-44.08) | 4.55 | 975.71 | Hilly and high altitude zone | Nilgiris | NIL | 180 | 2.40± 0.77 | 0.92(3) | 19.81 (16.22-32.25) | 67.91 (37.94-806.68) | 13.20 | 2828.57 | North western zone | Dhannapuri | DHA | 180 | 2.35±0.41 | 3.98(3) | 6.98 (3.76-10.93) | 24.45 (14.20-187.13) | 4.65 | 997.14 | | Krislmagiri | KRI | 180 | 1.33±0.31 | 2.66(3) | 9.51 (6.41-14.56) | 88.20 (40.46-643.18) | 6.34 | 1358.57 |
|
| Where; n: No. of larvae tested; Df: degrees of freedom; FL: Fiducial limits; RR1, Resistance Ratio - the LC50 of laboratory maintained susceptible population was utilized as factor divisor. RR2: Based on LC50 (0.007 ppm) of previous susceptible lab. Population was utilized as factor divisor | | Table 2.: Effect of different synthetic and natural synergists on the toxicity of cyantraniliprole to laboratory selected susceptible and highly resistant field populations of P. xylostella
| Population | Insecticide | n | Slope±SE | χ2(df) | LC50(ppm) (95% FL) | SR | NIL | Cyantranililprole | 180 | 2.395± 0.767 | 0.92(3) | 19.81 (16.22-32.25) | – | Cyantranililprole + DEM | 180 | 3.088± 0.641 | 0.38 (3) | 7.19 (5.25-8.60) | 2.76 | Cyantranililprole + PBO | 180 | 2.91± 0.72 | 0.51 (3) | 6.91 (4.25-8..55 | 2.87 | Cyantranililprole + TPP | 180 | 8.16± 2.04 | 0.95 (3) | 16.81 (14.04-18.16) | 1.18 | Cyantranililprole + TY | 180 | 5.95± 1.40 | 0.29 (3) | 14.34 (11.39-15.95) | 1.38 | Cyantranililprole + RO | 180 | 4.82± 1.19 | 1.75 (3) | 12.33 (9.11-14.04) | 1.61 | KRI | Cyantranililprole | 180 | 1.325± 0.309 | 2.66(3) | 9.51 (6.41-14.56) | – | Cyantranililprole + DEM | 180 | 2.60± 0.47 | 3.04 (3) | 2.85 (1.29-4.05) | 3.34 | Cyantranililprole + PBO | 180 | 1.92± 0.46 | 1.49 (3) | 4.79 (2.94-6.24) | 1.99 | Cyantranililprole + TPP | 180 | 2.32± 0.51 | 0.08 (3) | 8.33 (6.10-10.28) | 1.14 | Cyantranililprole + TY | 180 | 2.58± 0.57 | 0.56 (3) | 8.99 (7.22-10.87) | 1.06 | Cyantranililprole + RO | 180 | 2.38± 0.52 | 0.10 (3) | 8.60 (6.32-10.56) | 1.10 | Susceptible | Cyantranililprole | 180 | 1.31± 0.33 | 2.22(3) | 1.50 (0.95-2.23) | – | Cyantranililprole + DEM | 180 | 0.71± 0.17 | 1.83 (3) | 0.29 (0.13-0.60) | 5.17 | Cyantranililprole + PBO | 180 | 1.13± 0.20 | 2.74 (3) | 0.72 (0.45-1.15) | 2.08 | Cyantranililprole + TPP | 180 | 2.35± 0.41 | 3.01 (3) | 1.05 (0.54-1.50) | 1.42 | Cyantranililprole + TY | 180 | 3.28± 0.577 | 2.22 (3) | 1.33 (1.07-1.56) | 1.13 | Cyantranililprole + RO | 180 | 1.77± 0.31 | 4.03 (3) | 0.81 (0.24-1.40) | 1.85 |
|
| n, No of larvae tested, Df, degrees of freedom; FL, Fiducial limits; SR, Synergist Ratio - LC50 of cyantraniliprole without synergist/LC50 along with synergist. | |
| Acknowledgements Authors thank Department of Agricultural Entomology, Tamil Nadu Agricultural University, Coimbatore for providing research facilities and their technical support. Top | |
|
|
|