|
|
|
Studies on certain antibacterial peptides in goat polymorphonuclear cells Kumar Sandeep, Nandi S., More T., Kaur S. Division of Biochemistry, Indian Veterinary Research Institute Izatnagar–243122 (U.P.) Abstract The antibacterial cationic peptides of caprine polymorphonuclear (PMN) cells have not been studied. Hence the PMN cells were isolated from whole caprine blood and the granular cationic proteins and peptides therein were extracted with 0.2 M sodium acetate buffer (pH 4.0). The extract was fractionated on a Sephadex-G-50 exclusion column (25 × 550 mm) which resolved these proteins into four major fractions. The last protein fraction which contained peptides showed the greatest antibacterial potency against E. coli and S. aureus. The results indicated that the antibacterial components were highly active at the optimum pH of 7.2 and the minimum inhibitory concentration for 2 × 106 CFU of E. coli and S. aureus as assessed from the peptides content was 10 and 20 µg respectively. The minimum incubation time required for the lytic action against E. coli was shorter than that for S. aureus. The antibacterial agent caused bacteriolysis as judged from β-galactosidase release into the medium, which indicated the increased permeability or damage to the inner plasma membrane of the bacteria. Top | Polymorphonuclear (PMN) cells play a pivotal role in protecting higher organisms against microbial invasion. They destroy the invading microbes by two principal mechanisms. One is an oxygen dependent mechanism in which H2O2, O−2 and HOCl are involved (Lehrer and Ganz, 1990). The other non-oxidative, microbicidal activities are mediated by the concerted action of various cationic proteins such as the bactericidal permeability increasing protein (BPI), lactoferrin, CAP-57, and several small molecular weight peptides. In addition, enzymes such as lysozyme, collagenase and several neutral serine proteases (azurocidin, cathepsin G, elastase etc.) are also associated with oxygenindependent killing of the microbes (Lehrer and Ganz, 1990). These proteins are located in the granular fractions of the neutrophils. |
Major species differences exist between these oxygenindependent antimicrobial substances, e.g., cattle lack lysozyme and horses are devoid of defensins (Pellegrini et al., 1991). Gennaro et al. (1983a) reported that, in contrast to neutrophils of other species, bovine neutrophils contained, in addition to azurophilic and specific granules, a third larger and much denser type of granule, which contained cationic proteins that are unique to these granules, except for lactoferrin. |
As there are no similar reports on the PMN cells of goats, the antibacterial property of caprine PMN granular proteins and peptides was studied. |
Top Materials and Methods Isolation of PMN cells and acid extraction of granular proteins Peripheral blood was collected from the jugular vein of healthy goats using EDTA as an anticoagulant (1 mg/ml) and kept ice-cold while being brought to the laboratory. The PMN cells in the blood were isolated after hypotonic lysis of the RBCs followed by differential centrifugation (Carlson and Kaneko, 1973). A pool of 500 ml of blood yielded 109 cells that were >95% PMN cells. Typically 90-92% of these cells were PMN neutrophils. The PMNs were subjected to granular separation and were extracted with 0.2 M sodium acetate buffer pH 4.0 (Gennaro et al., 1983b). The extract was collected, lyophilized and stored at −20°C till further use. Goat PMN granular extracts were subjected to Acid Urea Polyacrylamide gel electrophoresis (AU-PAGE) and Sodium dodecyl sulfate Polyacrylamide gel electrophoresis (SDS-PAGE) (Roy et al., 1997). Size exclusion chromatography This was performed on the goat PMN granular extract according to Gennaro et al. (1983b). A column (25 × 550 mm) was packed with Sephadex G-50 (Pharmacia, Uppsala, Sweden) and equilibrated with the eluting buffer (0.2 M sodium acetate, pH 4.0). Seventy mg of the lyophilized acid extract in 2.0 ml of the eluting buffer was loaded on to the column and the fractionation was carried out at a constant flow rate of 18.0 ml/hr. Fractions of 3.0 ml were collected with an automatic fraction collector at 4°C and the absorbance at 280 nm was recorded. Each of the protein fractions indicated from the chromatographic record was pooled, desalted, lyophilized and tested for its antibacterial property. In vitro assay of the antibacterial activity of the fractions Pure cultures of E. coli and S. aureus obtained from the Bacteriology Division, Indian Veterinary Research Institute, were subcultured for 4 hrs and finally suspended in nutrient broth to give a standard O.D. of 0.80 at 600 nm in a spectrophotometer (Spectronic 2000) which represented 2 × 106 viable CFU/ml in a reference standard curve. The bactericidal activity was assayed in sterile tubes in 0.5 ml of incubation mixture containing 0.48 ml of sterile nutrient broth. In some experiments.0.48 ml phosphate buffered saline (PBS) was used to test the effect of nutrient agar on microbicidal activity of the peptides. E. coli and S. aureus (2.0 × 106 CFUs) were incubated with granular proteins in different tubes at 37°C with intermittent shaking for either 30 min or in some experiments up to 90 min. Each experiment included a tube containing an equivalent amount of bovine serum albumin (BSA) in place of granular proteins. At the end of the incubation, the assay mixtures were serially diluted upto 104 fold with PBS and 0.1 ml of the last three dilutions were plated onto nutrient agar plates. The numbers of colonies formed were counted after 36 h incubation. The bactericidal activity was then determined by recording the decrease in colony forming units (the mean from three experiments) in which the E. coli or S. aureus were incubated with goat granular peptides as compared to controls. The microorganisms were incubated in buffer with BSA only. Typically, the number of CFUs in the controls increased by 10-20% from the initial input of CFUs during the incubation at 37°C. Enzymatic assay (β-galactosidase) of lysis of E. coli The method described by Lehrer et al. (1989) was adopted. Briefly, the assay mixture contained 9 × 108 CFUs of E. coli in 1.0 ml of nutrient broth and 100 µg of the fractions. The control tube contained 100 µg BSA in place of the fraction. The tubes were incubated at 37°C for 0 to 90 min, centrifuged at 1500xg for 10 min and in 0.1ml of the supernatant was assayed for β–galactosidase activity. The estimation of activity of this cytosolic enzyme was carried out according to McFeters et al. (1967). Protein estimation Protein was estimated according to Lowry et al. (1951) using bovine serum albumin as the standard. Top Results AU-PAGE and SDS-PAGE of goat PMN granular acetate extracted cationic proteins and peptides are given in Fig. 1 and 2. AU-PAGE revealed presence of peptides with maximum cationicity and nearer to lysozyme, while SDS-PAGE separated proteins based on their mass, which in the presence study showed 6 bands in the range of 4 to 1.5kD.
|
Size exclusion chromatography The acid extract of goat PMNs on the Sephadex G-50 column yielded four major fractions (Fig. 3). The figure shows minor second peak, which however was considered part of first major peak while pooling the eluted fractions. The high molecular weight proteins were eluted in the void volume whereas the last fraction contained the peptides.
Bactericidal assays When the four major fractions were tested for their antibacterial assay against E. coli and S. aureus, the highest activity was found in fraction IV, followed by fraction III (Table 1). All the subsequent studies were carried out with fraction IV. A pH of 7.2 was optimum for the antibacterial activity of this fraction (Table 2). When fraction IV was incubated with E. coli or S. aureus in PBS,there was no appreciable antibacterial effect but this was marked when nutrient broth was added to the incubation medium (Table 3). This indicated the bactericidal action of the peptide to be nutrient dependent.
The minimum inhibitory concentration (MIC) of fraction IV when incubated for 30 min with 2.0x106 CFUs of E.coli and S.aureus was 10 and 20 µg respectively (Table 4). The bactericidal effect increased sharply after 10 min incubation in the case of E. coli and 30 min in the case of S. aureus (Table 5). The amount of ß-galactosidase in the incubation medium indicated that bacteriolysis attained its maximum level after 60 min of incubation of E. coli with fraction IV (Table 6).
Top Discussion Presence of goat PMN granular cationic peptides was confirmed by both AU-PAGE as well as SDS-PAGE. The results are similar to the reports published in buffalo (Roy et al., 1997). |
Antibiotic peptides are key components of innate immunity. Their distribution throughout the animal kingdom is ubiquitous reflecting the importance of these molecules in host defense (Zasloff, 1992). Among the best studied of these peptides are mammalian defensins. Although defensins were discovered initially in neutrophils and monocytes granules, they have more recently been identified as a part of antimicrobial barrier of the mucosal surface (Jones and Bevins, 1992). ß defensins are cationic, cysteine rich molecules and most have broad-spectrum antimicrobial activity against bacteria, fungi, viruses (Zasloff, 2002). β-defensins contribute to a protective barrier on mucosal surfaces including tongue, nasal, intrapulmonary airways and small intestine (Mathews et al., 1999). Cattle express at least 15 different β-defensin genes including two found in epithelial tissues, tracheal antimicrobial peptide (TAP) and lingual antimicrobial peptide (LAP) (Diamond et al., 1991; Schonwetter et al., 1995). Several diseases in humans and laboratory animals are characterized by impairment in the function of antimicrobial peptides (Zasloff, 2002). There is also considerable data indicating epithelial defensins are upregulated in response to infections (Diamond et al., 1996). In cultured epithelial cells, TAP message is induced in response to lipopolysaccharide exposure. |
Stolzenberg et al. (1997) investigated the distribution and expression of ß defensin class in normal cattle and in animals in varying states of disease and proposed that expression of antimicrobial peptide is an integral component of the inflammatory response. The observation that last peak which contained the smallest proteins such as peptides was associated with the highest bactericidal potency against E. coli and S. aureus is compatible with the reports of Selsted et al. (1984), Ganz et al. (1985) and Marzari et al. (1988) in rabbits, humans and cattle, respectively. As has been shown by other authors, the antimicrobial effects of these goat PMN peptides were strongly pH dependent, the pH optimum being 7.2. Gennaro et al. (1983b) recorded that the bovine neutrophil granular proteins were very active in vitro in media of physiological pH and containing mono and divalent cations. Selsted et al. (1984) observed that purified antibacterial peptides from rabbit granulocytes NP-1, NP-2, NP-3a, NP-3b, NP-4 and NP-5 were best expressed at around neutral pH under conditions of low ionic strength. However, purified azurocidin showed its maximum antibacterial activity at pH 5.5 (Campanelli et al., 1990). Obviously there is variation in the pH optima for the antimicrobial activity of different PMN cationic proteins or peptides in different animals. As far as in vivo relevance of pH is concerned, surprisingly human PMN phagocyte vacuoles were not found to be strongly acidic, and studies with fluorescent pH probes showed that the newly formed phagosomes were neutral to mildly alkaline for the first 15 min, with subsequent acidification to pH 6.0 (Segal et al., 1981; Mayer et al., 1989). Accordingly the present observation that a neutral pH allowed the peptides to display optimal antibacterial activity may be extrapolated to in vivo conditions. Similar observations regarding the requirement for nutrients were recorded by Ganz et al. (1985). They reported that human defensins killed S. aureus, Pseudomonas aeruginosa and E. coli effectively in vitro when tested in 10 mM phosphate buffer containing certain nutrients, but had little or no bactericidal activity in nutrient free buffer. Lehrer et al. (1989) reported that when stationary phase E. coli ML-35 were exposed to human PMN peptides no β–galactosidase was released for >90 mn. In contrast, when these organisms were exposed to defensins in buffer containing nutrient (Sodium phosphate buffer-tryptic soy broth), hydrolysis of ONPG occurred, signifying that the inner membrane of the bacteria had become permeable. We also observed that the gram positive S. aureus required a higher concentration of the factor IV to check its growth, compared to E. coli. Weiss et al. (1980) observed that rough strains of S. typhimurium were more sensitive to the bactericidal, membrane-active cationic proteins from human and rabbit polymorpho-nuclear leukocytes, than smooth strains. Marzari et al. (1988) reported that the more cationic bovine PMN peptides of 4-8 kDa inhibited the growth of 5 × 104 CFU of E. coli at a MIC of 12-50 µg/ml. In addition a 1.6 kDa, highly cationic peptide also inhibited the growth of S. aureus and S. epidermis at MICs of 3-8 µg/ml. |
Our attempts to find out the minimum incubation period for utmost bactericidal effect of the peptides revealed that the bacterial viability decreased sharply after 10 min incubation in case of E. coli whereas viability declined sharply after 30 min in case of S. aureus. Our finding of increased β-galactosidase in the incubation medium after incubation of the E. coli cells with fraction IV suggested increased permeability in the inner and outer membranes. Lehrer et al. (1989) reported similar findings of inner and outer membrane permeabilization when E. coli bacteria were treated with human defensins. Because of their cationic nature these peptides adhere to the negatively charged surfaces of their targets and act as antibiotics by disturbing the membrane permeability in the microorganisms by opening voltage gated channels (Martin et al., 1995). |
It is concluded that caprine PMN cells also contain antimicrobial agents, which probably play a significant role in the immunodefence system. |
Top Figures Fig. 1: AU-PAGE pattern of acid extract of goat PMN cells. Lane N: Acid extract of goat PMN cell; Lane M: Lysozyme and lactoferrin run as standard
| 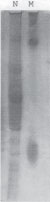 | |
| Fig. 2: SDS-PAGE of acid extract of goat PMN cells. Lane C: Acid extract of goat PMN cell; Lane M: Molecular weight markers
| 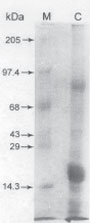 | |
| Fig. 3: Molecular exclusion chromatography of cationic proteins and peptides of goat PMN cells by using Sephadex G-50.
| 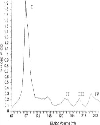 | |
|
Tables Table 1: In vitro antibacterial potencies of different Sephadex G-50 fractions of goat PMN granular proteins against E. coli and S. aureus
| Granular protein (10µg) | % killing efficiency |
| E. coli | S. aureus |
| Fraction I | 16.34 | 8.25 | Fraction II | 27.51 | 2.02 | Fraction III | 85.70 | 64.50 | Fraction IV | 97.99 | 94.25 |
| | Table 2: Effect of pH on the antibacterial activity of goat PMN peptides (10 µg of the peptides were incubated with 2.0x106 CFU of E. coli
| pH | % Killing efficiency |
| 6.5 | 32.50 | 6.8 | 64.61 | 7.0 | 69.64 | 7.2 | 78.63 | 7.5 | 48.57 |
| | Table 3: Effect of nutrients on the antibacterial activity of the goat PMN peptides (10 µg of the peptides were incubated with 2.0x106 CFU of E. coli and S. aureus).
| Nutrient | % killing efficiency |
| E. coli | S. aureus |
| PBS | 5.16 | nil | PBS+nutrient agar | 98.33 | 58.94 |
| | Table 4: Determination of minimum inhibitory concentration of the goat PMN antibiotic peptide (with 2.0x106 CFU of E. coli and S. aureus).
| Peptide | % killing efficiency Concentration |
| E. coli | S. aureus |
| 5 μg | 69.11 | 37.68 | 10 μg | 97.63 | 65.07 | 20 μg | 98.43 | 90.11 | 30 μg | 99.12 | 91.40 | 40 μg | 99.46 | 96.81 | 50 μg | 99.88 | 97.04 | 80 μg | 99.90 | 99.78 |
| | Table 5: Determination of minimum incubation time required for the optimum lytic activity of 10 and 20 µg of the peptides against E. coli and S. aureus. (2.0x106 CFU of both)
| Incubation time (min.) | % killing efficiency |
| E. coli | S. aureus |
| 10 | 4.01 | 4.35 | 20 | 44.45 | 17.34 | 30 | 65.72 | 31.46 | 40 | 85.67 | 52.20 | 60 | 95.23 | 68.28 |
| | Table 6: Kinetics of E. coli lysis as determined by the release of β-galactosidase in the incubation medium with 10 µg of the fraction IV
| Incubation time (min.) | Absorbance at 410 nm |
| Control | E. coli (9×108 cfu/ml) |
| 10 | 0.75 | 0.75 | 20 | 0.79 | 1.05 | 30 | 0.88 | 1.46 | 40 | 0.89 | 1.71 | 50 | 0.93 | 2.23 | 60 | 1.15 | 3.87 | 80 | 1.05 | 4.02 | 90 | 1.56 | 4.03 |
| |
| Acknowledgements The authors are grateful to the Director, IVRI for providing the necessary facilities and financial assistance. Top References | | CampanelliD., DetmersP.A., NathanC.F., GabayG.E.
(1990).
J. Clin. Invest.,
85:
904. TopBack | | CarlsonG.P., KanekoJ.J.
(1973).
Proc. Soc. Exp. Biol. Med.,
142:
240. TopBack | | DiamondG., RusselJ.P., BevinsC.L.
(1996).
Proc. Natl. Acad. Sci., USA.,
93:
240. TopBack | | DiamondG., ZasloffM., EckH., BrasseurM., Lee MaloyW., BevinsC.L.
(1991).
Proc. Natl. Acad. Sci.,USA.,
88:
240. TopBack | | GanzT., SelstedM.E., SzklarekD., HarwigS.S.L., DaherK., BaintonD.F., LehrerR.I.
(1985).
J. Clin. Invest.,
164:
240. TopBack | | GennaroR., DewaldB., HorishbergerU., GulberH.U., BaggioliniM.
(1983a).
J. Cell Biol.,
96:
240. TopBack | | GennaroR., DolzaniL., RomeoD.
(1983b).
Infect. Immun.,
40:
684. TopBack | | JonesD.E., BevinsC.L.
(1992).
J. Biol. Chem.,
267:
240. TopBack | | LehrerR.I., GanzT.
(1990).
Blood,
76:
240. TopBack | | LehrerR.I., BartonA., DaherK.A., HarwigS.S.L., GanzT., SelstedM.E.
(1989).
J. Clin. Invest.,
84:
240. TopBack | | LowryO.H., RosebroughN.J., FarrA.L., RandallR.J.
(1951).
J. Biol. Chem.,
193:
240. TopBack | | MartinE., GanzT., LehrerR.I.
(1995).
J. Leuk. Biol.,
58:
240. TopBack | | MarzariR., ScaggianteB., SkerlavajB., BittoloM., GennaroR., RomeoD.
(1988).
Infect. Immun.,
56:
240. TopBack | | MathewsM., JiaH.P., LoshG., ScotM.G., TackB.F.
(1999).
Infect. Immun.,
67: (6)
240. TopBack | | MayerS.J., KeenP.M., CravenN., CravenN., BourneF.I.
(1989).
J. Leuk. Biol.,
45:
240. TopBack | | McFetersG.A., SandineW.E., EllikerP.P.
(1967).
J. Bacteriol.,
93:
240. TopBack | | PellegriniA., WaiblingerS., Von FellenbergR.
(1991).
Vet. Res. Commun.,
15:
240. TopBack | | RoyS.C., SinghV.K., MoreT.
(1997).
Vet. Res. Commun.,
21:
240. TopBack | | SchonwetterB.S., StolzenbergE.D., ZasloffM.A.
(1995). Science,
267:
240. TopBack | | SegalA.W., GeisowM., GarciaR., HarperA., MillerR.
(1981).
Nature,
290:
240. TopBack | | SelstedM.E., SzklarekD., LehrerR.I.
(1984).
Infect. Immun.,
45:
150. TopBack | | StolzenbergE.D., AndersonG.M., AckermannM.R., WhitlockR.H.
(1997).
Proc. Natl. Acad. Sci. USA.,
94:
240. TopBack | | WeissJ., Beckerdite-QuagliateS., ElsbachP.
(1980).
J. Clin. Invest.,
65:
240. TopBack | | ZasloffM.A.
(1992).
Curr. Opin. Immunol.,
4(1):
240. TopBack | | ZasloffM.A.
(2002).
New Eng. Med J.,
347:
240. TopBack |
| |
|
|
|